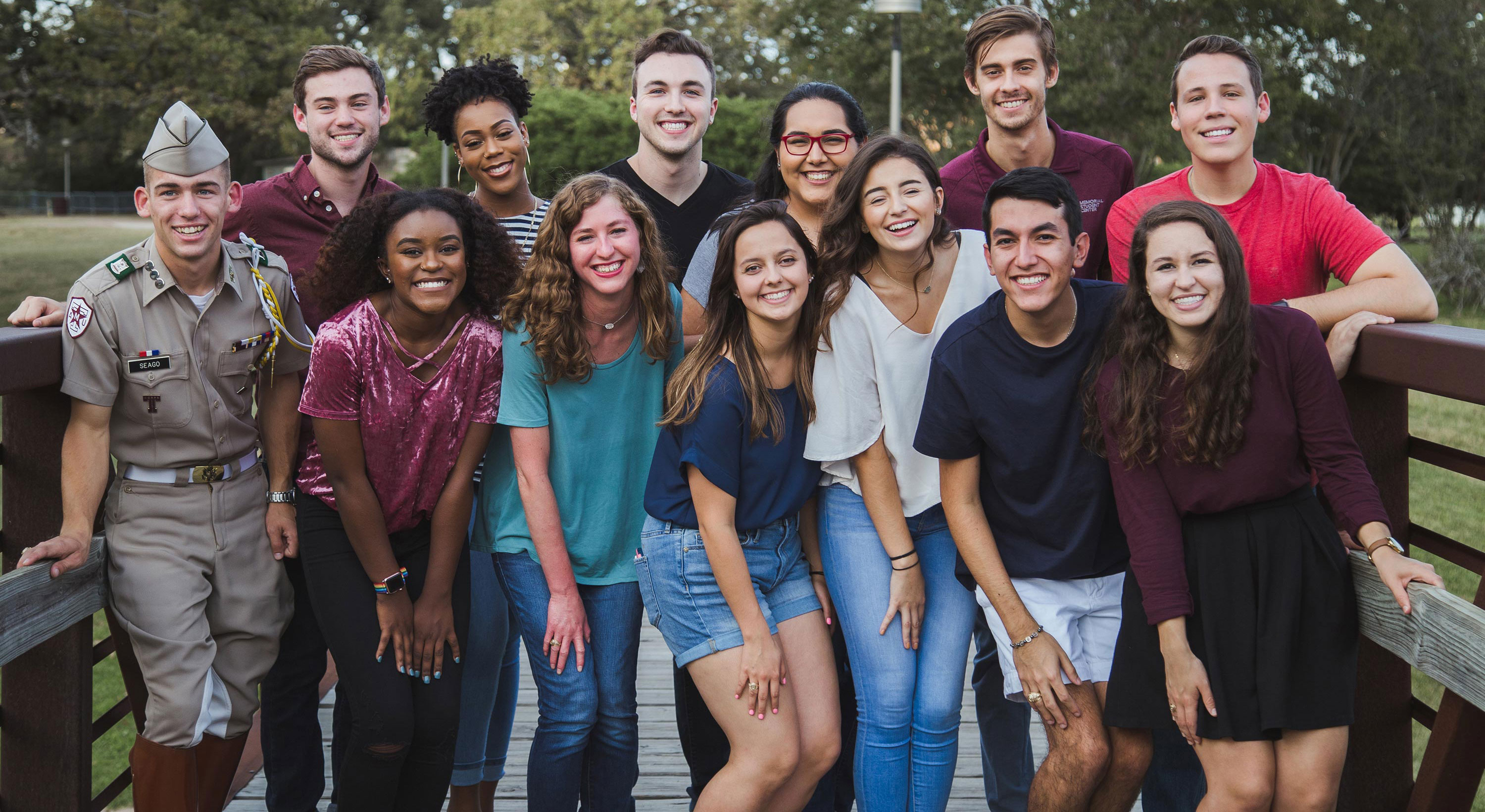
Homepage
Featured Items
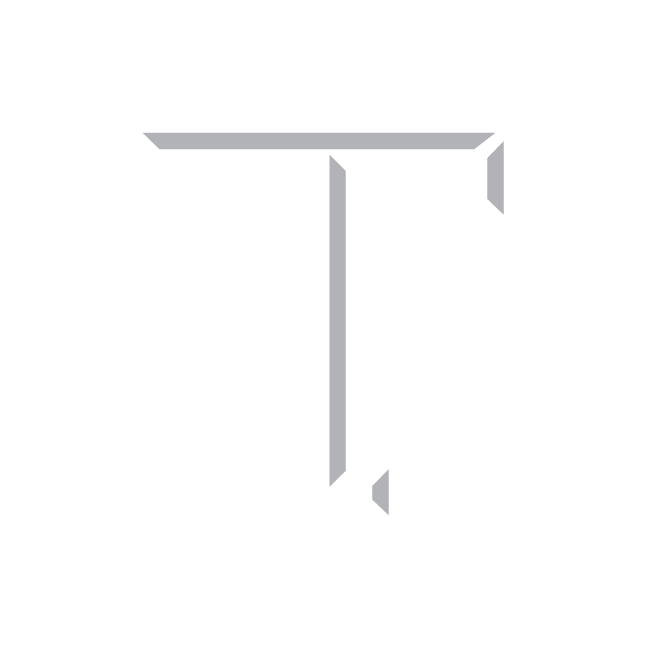
There's a place for you!
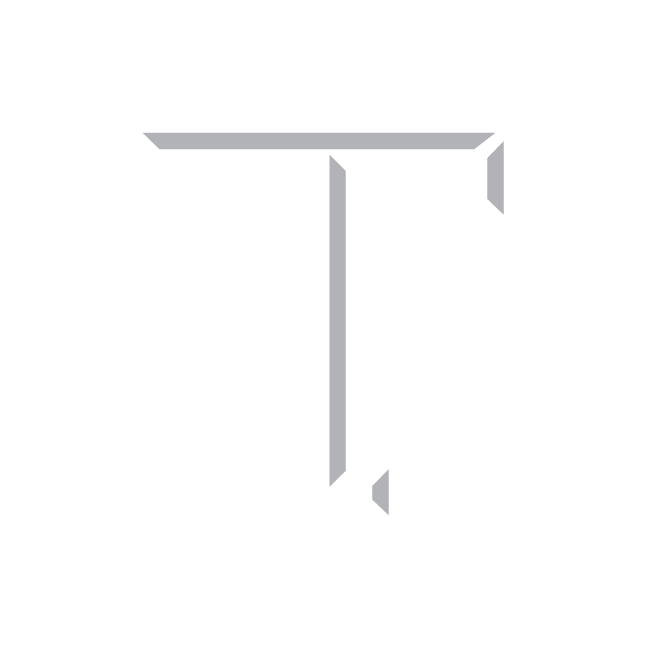
Memorial Student Center
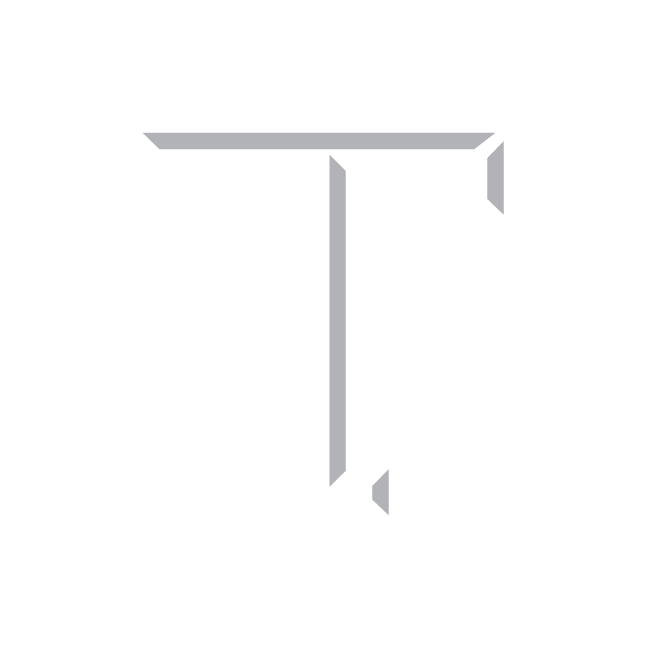
Event and Concessions Forms
Events
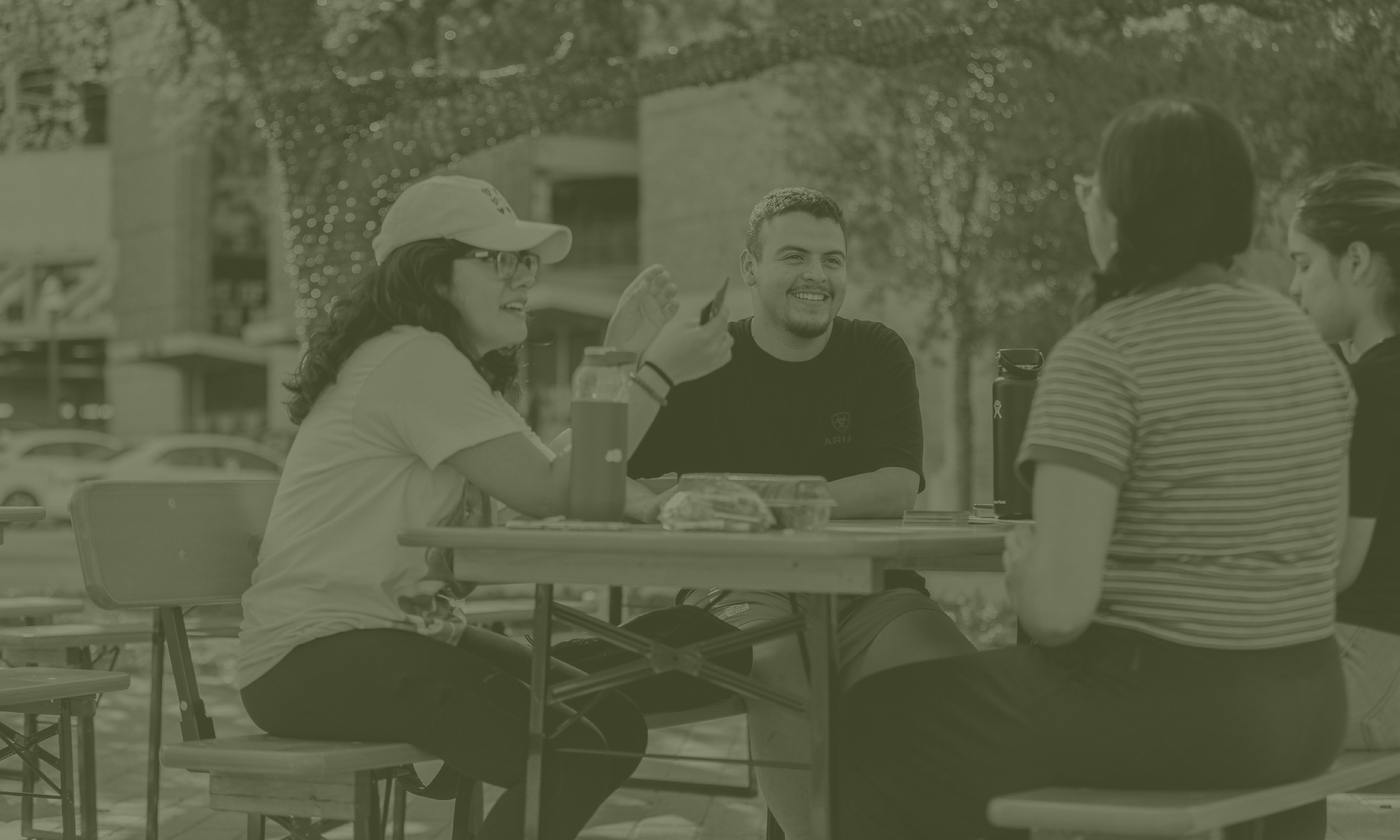
Mi Casa es Su Casa
MSC CAMAC
Fri, Apr 26, 2024 5:00 pm
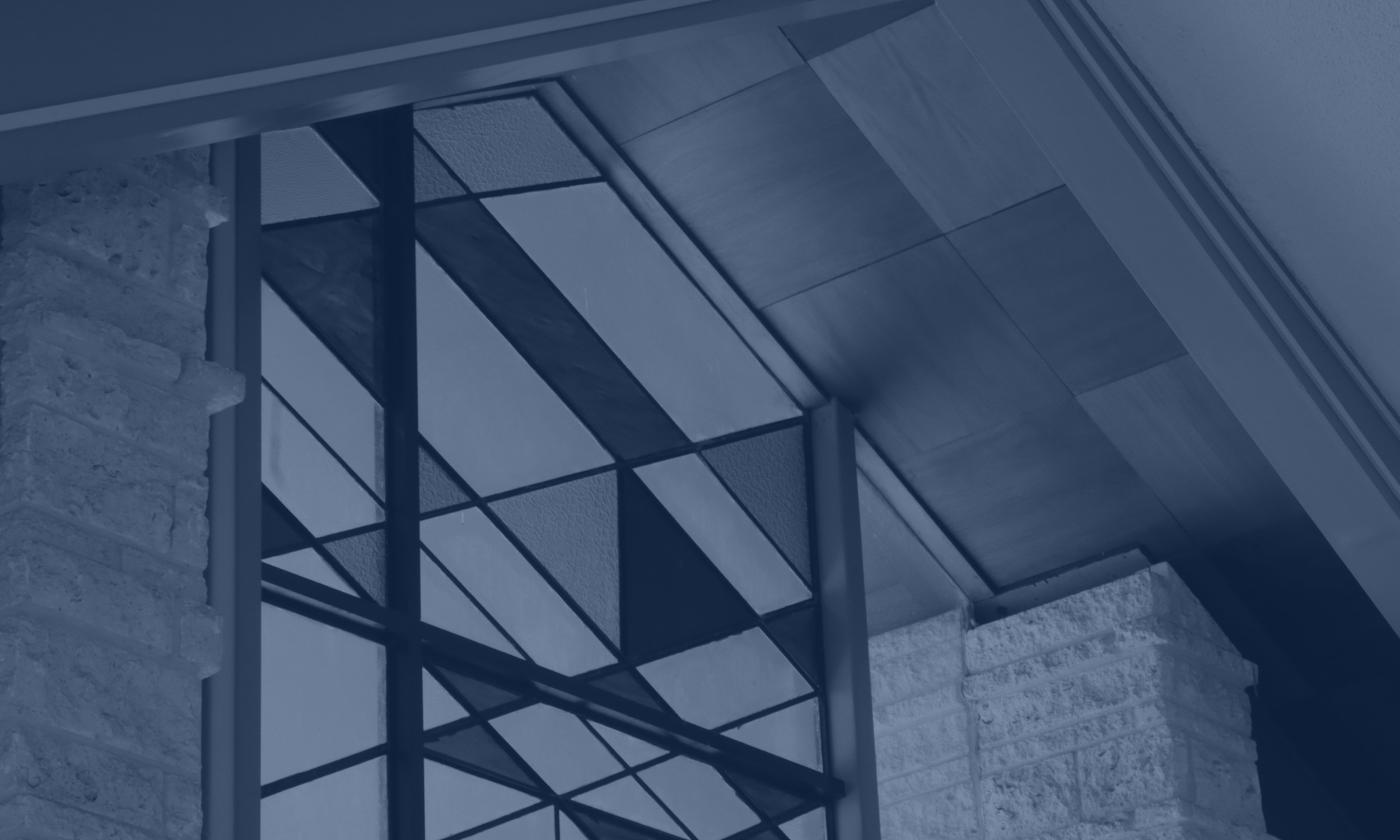
Epic Large Group
Epic Movement
Fri, Apr 26, 2024 7:00 pm
All Faiths Chapel
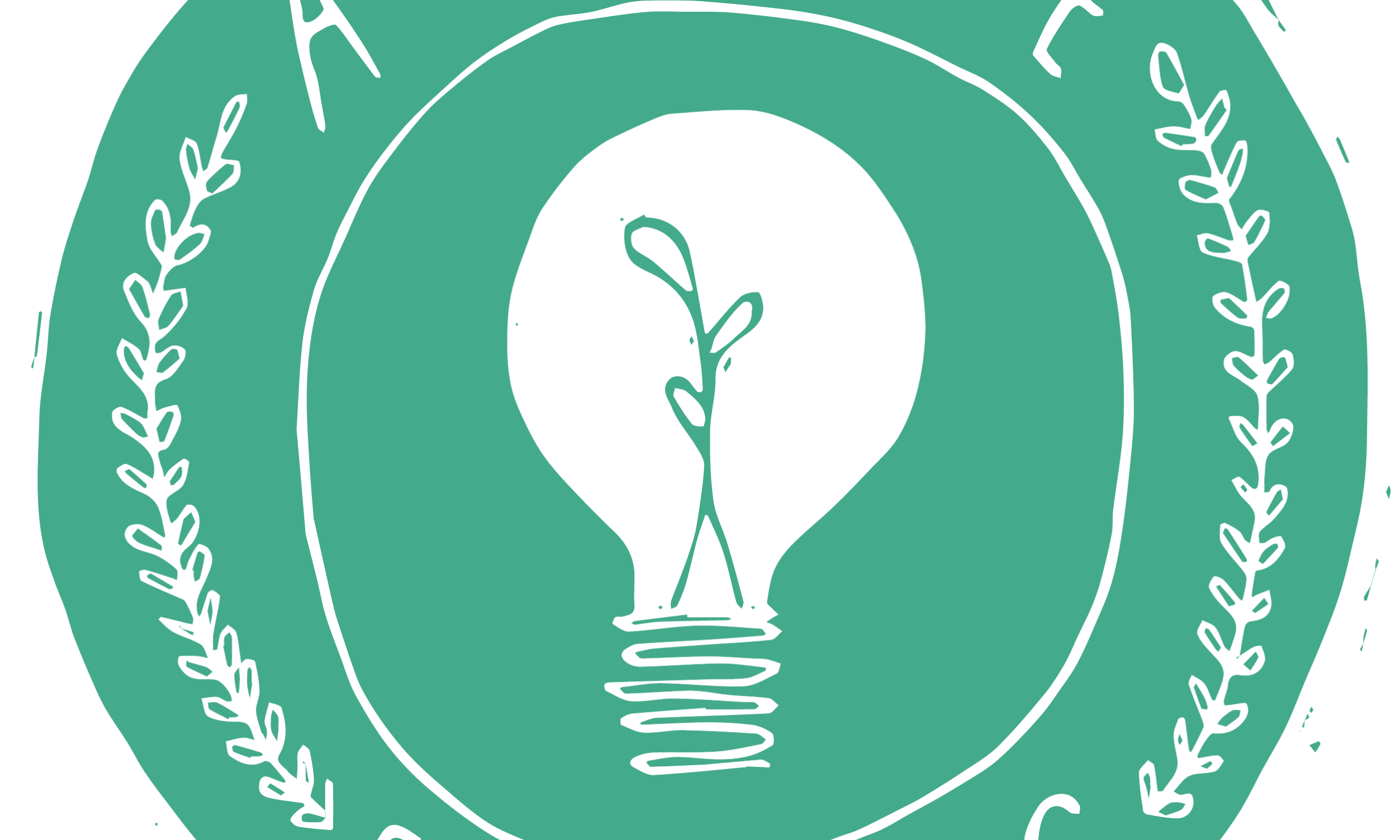
Earth Day 5K Fun Run
Aggie Eco-Representatives
Sat, Apr 27, 2024 8:00 am
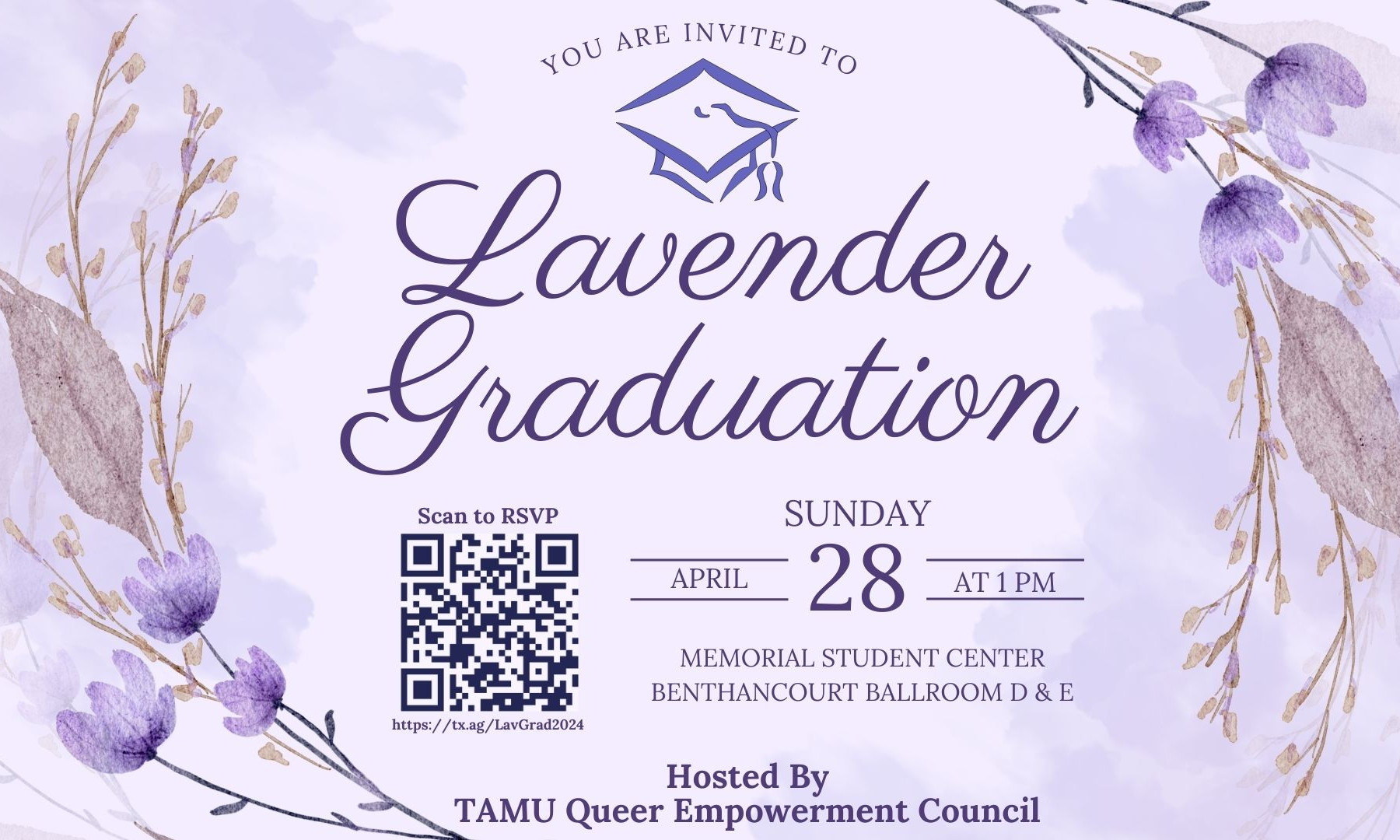
Lavender Graduation
Queer Empowerment Council
Sun, Apr 28, 2024 1:00 pm
Memorial Student Center